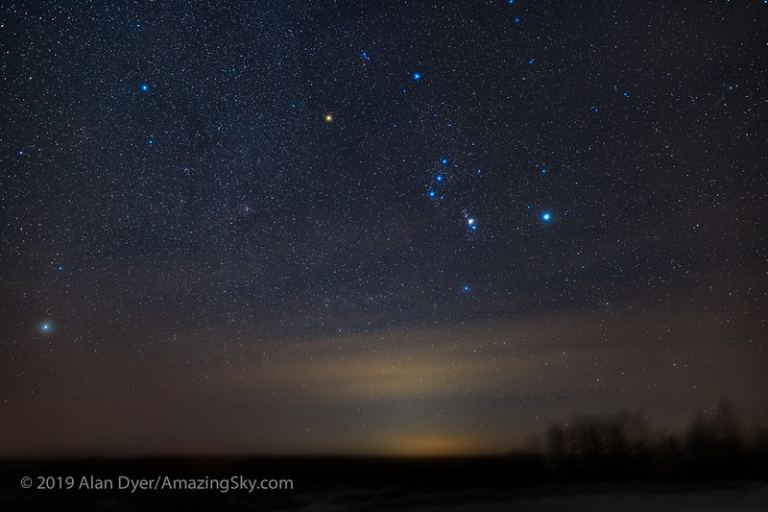
Betelgeuse: The Life (and Death?) of Supergiant Variable Stars
Most of you know me as a weather enthusiast. But throughout my life, I’ve also had a keen fascination with astronomy. My grandpa first introduced me to the topic when I was about 4 or 5 years old. I learned the names of the (then nine) planets, and the basic features of each world. By the time I was in fourth grade, my interest was budding into a real hobby: there were many family trips to Goldendale Observatory, back in the early to mid-1990s.
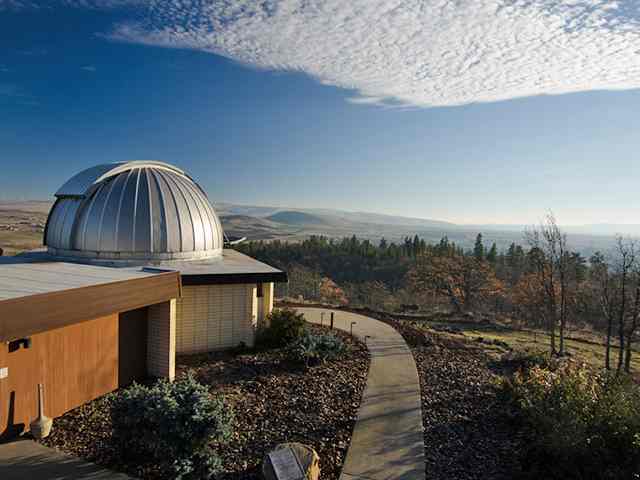
Among my most memorable events over the years have been: the Shoemaker-Levy comet crash with Jupiter in 1994; the close approach of the Hyakutake comet in 1996; close approach with Mars in 2003; the international “Year of Astronomy” in 2009; and of course the total solar eclipse of 2017.
But none of those events would compare to what the space & astronomy media have been buzzing about this past month: speculation that a relatively nearby star may be about to explode in a supernova. I’m referring of course to Betelgeuse, the red supergiant star in the upper-left corner of the constellation Orion the hunter. Almost every day on my Google news feed, another article pops up about the subject, so I figured this would be a good time to enlighten my fellow weather and astronomy geeks as to what is going on.
Take the term “may be about to explode” with a grain of salt: most astronomers are not actually expecting a Betelgeuse supernova in the immediate future. Most stellar models suggest the star has at least a 100,000-year window during which it might explode – and it’s very hard to tell if its time to go will be next month or millennia from now. The reason for the speculation is that Betelgeuse is a variable star which sees its brightness fluctuate over periodic intervals. However, over the last few months the star has dimmed somewhat more dramatically than it normally does. And while a ‘super-dimming’ might occur in the run-up to a supernova, there are many other explanations that are just as plausible.
=====
THE GIANT’S SHOULDER
Like many stars in the night sky, Betelgeuse was named by Arabic astronomers during the Middle Ages. Its name means “the giant’s shoulder,” and it lies in the upper-left corner of Orion. The other bright star, Rigel, is in the lower-right corner. (Things are a little different in the Southern Hemisphere, where constellations are reversed top-to-bottom!)
It is a red supergiant star near the end of its lifespan. There is wide debate on the mass; which could range from 10 times that of the sun to as much as 20 times. However, at roughly 1.2 billion km (760 million miles) in diameter, it is more than 900 times as “fat” as the sun and has over half a million times the volume. If placed at the center of the solar system, its surface would come very close to Jupiter’s orbit! Stars this large almost always end their lives with a supernova explosion. Unfortunately for astronomers, there is no known method for telling when a red supergiant’s end is drawing nigh.
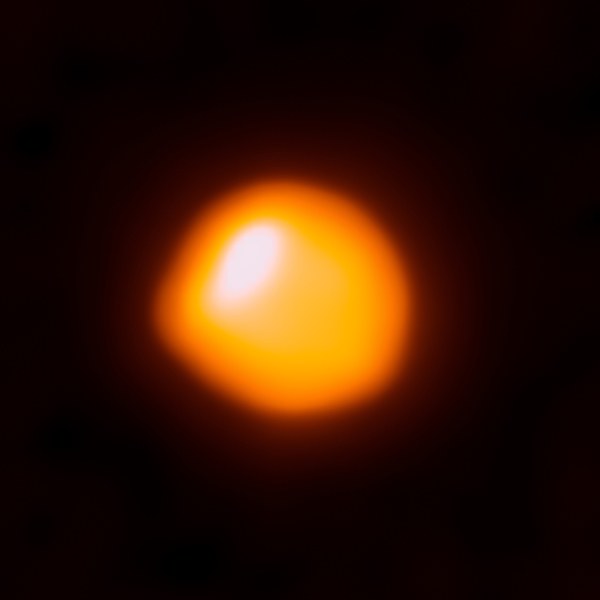
One feature of Betelgeuse, however, which can be readily observed is its periodic fluctuation in brightness. Large, senile stars like this tend to be dynamically unstable, due both to their highly fast-paced core fusion reactions and the fact that the outer layers are very diffuse. The variability of Betelgeuse has been monitored since at least the 1830s. Observers noticed that at peak brightness, Betelgeuse is similar to the neighboring ‘winter stars’ of Rigel, Procyon and Capella. But when dim, the red star can be fainter than Aldebaran, which lies to the west of Betelgeuse in the constellation Taurus.
In the 1920s, with the help of an interferometer, the diameter of Betelgeuse’s disk was measured for the first time. It is one of only a few stars which are both close enough and large enough, to be resolved in this manner.
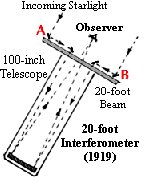
VARIABLE STARS
Astronomers have been observing the patterns of variable stars for centuries. They have proven invaluable tools in determining distances to remote galaxies, and also have helped shed light on fundamental questions concerning the age and motion of the universe.
Some stars vary in brightness due to extrinsic factors: two stars in a binary system may eclipse one another, resulting in a temporary drop in observed brightness. A large planet crossing in front of a star can also affect brightness. So can clouds of gas and dust in the vicinity.
But many stars are intrinsically variable, meaning that the temperature, luminosity and diameter of the star itself fluctuate. This pulsation can be either totally random, or regular and periodic. In the case of Betelgeuse, the pattern is semi-regular: there is a primary pulsating cycle every 425 days, with several less-regular cycles occurring inside the main one.
One of the first and most famous periodic variable star types to be studied were the Cepheids. They pulsate in a highly rhythmic pattern, with periods ranging from days to months. By knowing both the period and the apparent brightness of the Cepheid, its distance can be calculated – and it is in this way that early measurements of the Andromeda Galaxy were taken.
Many, if not most, red giants and supergiants become variable at some point. The variation can either be highly regular, in the case of ‘Mira’ variables, or semi-regular in the case of stars like Betelgeuse.
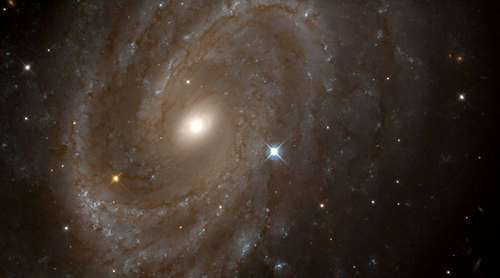
THE AGING OF STARS
Why do massive stars tend to pulsate late in their lives? The key lies in what is happening inside the star. For most of a star’s life, called the “main sequence,” the thermodynamics of the stellar interior are in equilibrium; fusion reactions in the core are steady in their output, and the thermal pressure of the core exactly balances the gravitational pressure of the star’s mass. (To be sure, fusion reactions and energy output do gradually accelerate over the star’s life, but these changes occur gradually on a scale of many millennia, not months or years.)
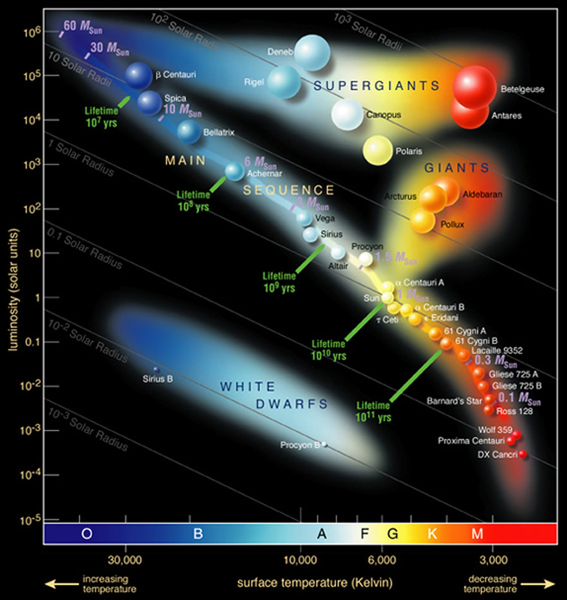
But once all the hydrogen in the core has been consumed and fused into helium, the star’s inner dynamics are thrown out of whack. Deprived of thermonuclear output, the core of the star begins to collapse under its own weight. Eventually this generates enough heat to ignite an envelope of hydrogen surrounding the core. This second ignition of fuel causes the outer layers of the star to expand dramatically. As they expand, the temperature cools and the star appears to shine red. This is the red giant (or supergiant) phase.
What happens next depends on the mass of the star. A star similar in size to the Sun will eventually fuse helium into carbon and a small amount of oxygen, in a rapid reaction called the “triple alpha process” or “helium flash.” But the core never gets hot and dense enough to start the heavier fusion reactions, and as a result the extremely dense and small core remains as a ball of inert carbon called a white dwarf.
Stars bigger than about 8 solar masses, however, do become hot enough to fuse carbon and oxygen into heavier nuclei, such as magnesium and silicon. These two can subsequently fuse to form iron and nickel. But once iron is formed in the core, no more fusion reactions are possible. This is because iron nuclei are more tightly bound to each other than almost any other atom. “High binding energy” can best be thought of as an analogy to low potential energy or low enthalpy: once the nucleus is bound so tightly, you have to add energy from outside in order to force any further reactions. This is when the final collapse of the core begins: with no more thermonuclear pressure holding back gravity, everything shrinks into a ball of neutrons perhaps 10 kilometers wide, and weighing billions of tons per spoonful.
The collapse releases so much energy that the outer layers are blown away in a cataclysmic explosion, called a Type II supernova. (Type I supernovae are generally caused by white dwarfs that attract too much foreign matter and collapse under their own weight.)
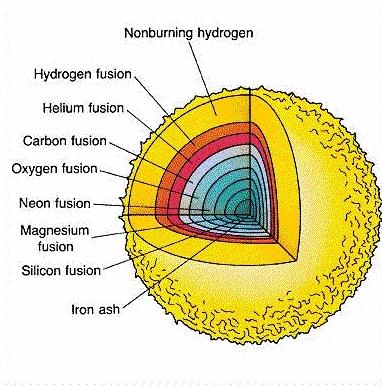
What does all this have to do with variable stars? During these final stages of fusion, the internal dynamics of the star are thrown into flux: new reactions are starting and stopping on a frequent basis, and the total energy output (i.e. wattage) of the star is thousands of times higher than when it was younger. The outer layers don’t know how to deal with this erratic behavior in the core. They respond by pulsating, by distorting the star into unusual shapes, and sometimes, by expelling a chunk of the star into space. (Indeed, imagery of Betelgeuse has revealed a series of faint nebulas in the vicinity; most likely caused by the supergiant star’s occasional bouts of flatulence.)
THE SUPERNOVA HYPE
Why did everyone start talking about Betelgeuse a little more than a month ago?
Astronomers have been recording the star’s variability for nearly two centuries. In that time, the star has generally fluctuated between +0.3 and +1.3 magnitude; the higher the number, the fainter the star appears in the night sky. Each order of stellar magnitude corresponds to a factor of 2.5 in total light reaching the observer’s eye.
Around October of 2019, observers noticed Betelgeuse dimming – and dimming quite rapidly. It seemed to be happening more suddenly than the historical record would lead them to expect. By late December the star had fainted to +1.3 magnitude, all in a matter of just three of four months. Scientific and space publications began to publish articles about the somewhat unusual behavior.
Almost immediately, news outlets across the Internet lit up with sensationalized hype about Betelgeuse and supernovas. Part of it stems from the fact that the star will explode some time in the next 100,000 years; we just don’t know when. But at least part of it has to do with the nature of today’s media in general, and its appetite for “clickbait.” (Those of you who have followed the news in advance of a possible snow storm in the Pacific Northwest, know what I’m getting at!). And of course, the fact that a supernova less than 700 light-years away would be a historically dramatic event, only threw fuel onto the flames of speculation.
In the month since the story broke, Betelgeuse has dimmed further to +1.5 magnitude, which is the lowest it has been in modern history. But the dimming process itself has been slowing down recently, perhaps a sign that the giant star is approaching the bottom of its current “super-minimum.”
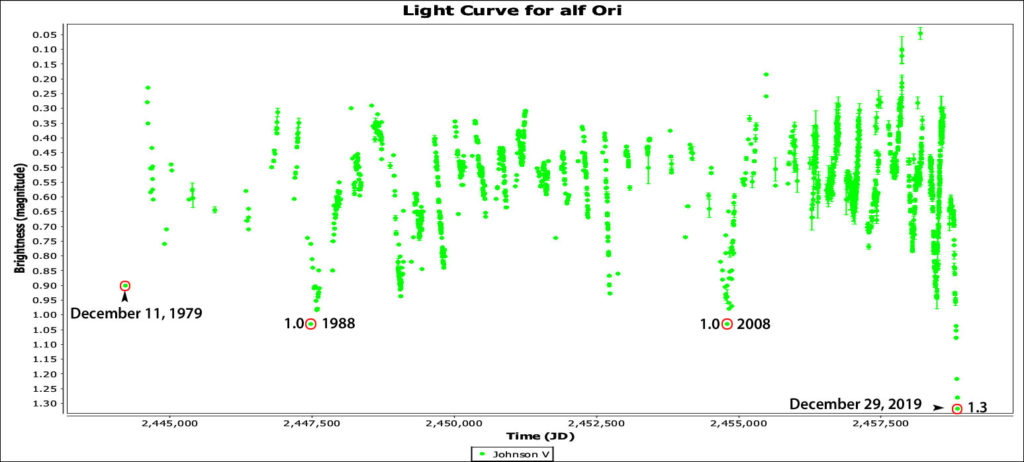
In addition to the “primary” 425-day cycle, Betelgeuse also has a more subtle oscillation on a 5.9-year cycle. It causes both the primary minima and primary maxima to migrate up and down every few cycles. Smaller and less regular cycles occur on a 100-180 day basis, as well. These have combined to cause a diverse range of variations over the decades. But none of the fluctuations have been as pronounced as what we’ve seen in the past four months.
=====
So…what do I think is really happening? It could be that several of Betelgeuse’s variable cycles are coming into the minimum phase simultaneously. Kind of like how several wave pulses in an ocean can phase up together and create a “sneaker wave,” in the same manner we could be seeing the short, medium, and long-term cycles all bottoming out at the same time. The short-term cycles, in addition, have been known to vary in amplitude – perhaps the current short cycle is a little deeper than usual.
In addition, Betelgeuse is known for ‘farting’ some of its material into space at times. These clouds of cosmic funk tend to remain in orbit around the host star for quite some time. Gas clouds have a habit of dimming whatever light passes through them. What if, at the same time all of Betelgeuse’s normal variable cycles were in minimum phase, a recently expelled star fart were to pass directly in front of the supergiant, as observed from Earth?
When you add up such a perfect storm of events – all variable cycles in faint phase; deeper-than-normal short term cycles; plus a bunch of gas blocking our view from Earth – it’s not inconceivable that you could get the unusual +1.5 magnitudes we’ve been seeing this month.
Assuming all or most of this is true, then we should expect to see Betelgeuse’s brightness rebound over the next several months, as the overlapping faint cycles end and the star farts drift out of the way. If, on the other hand, the star stays extremely dim for another 6-12 months (or becomes even dimmer still), then it would be a clear sign that something more significant is afoot. But even then, it may not be the red supergiant’s final death knell.
As mentioned above, supergiant stars go through many different stages of core fusion late in their lives: carbon to oxygen to neon to magnesium; then on to silicon, sulfur and iron. Every once in a while, one stage of burning will end and another stage will commence. When this happens, the energy output and brightness of the star can change in a fundamental way. If Betelgeuse’s luminosity stabilizes at a new, fainter long-term level than before (or perhaps, brighter than before!), it might be a sign that the star has turned a corner into the next phase of its evolution.
JUST FOR FUN…
Let’s finish by asking the inevitable question: What if Betelgeuse were to actually go supernova in the near future?
Throughout human history there have been only seven recorded supernova events within our own galaxy. The oldest was recorded in the year 185 A.D. by Chinese astronomers, and the most recent was in 1604 – about one visible explosion every 300 years, on average. Yet at the same time, stellar formation models suggest that a galaxy our size ought to have one such explosion every fifty years. (My guess is that many of the supernovae that explode on the opposite side of the Milky Way, might have been too faint for human observers to have traditionally recognized.)
It was the explosion of 1572, analyzed by Tycho Brahe, which originally gave rise to the name supernova; Brahe named the “new” star in Cassiopeia as a nova. The “super” prefix came about only in the 20th century, once the theories of stellar evolution and astrophysics were better understood. (What we now call a regular “nova” is caused by foreign matter falling into a white dwarf or neutron star, and heating up explosively before being ejected.)
The most famous observed supernova occurred in 1054, and was responsible for the formation of the Crab Nebula in the constellation Taurus. This explosion occurred nearly 6,300 light-years from Earth, and was visible in daylight for at least a couple months. It is believed to have been approximately magnitude -6; roughly five or six times the brightness of Venus. It was not until at least 1731, however, that the remaining nebula was finally observed in a small telescope. French astronomer Charles Messier studied the nebula in 1758, and it became the first of his famous “Messier Objects” which, to this day, remain the most popular sky targets for amateur astronomers.
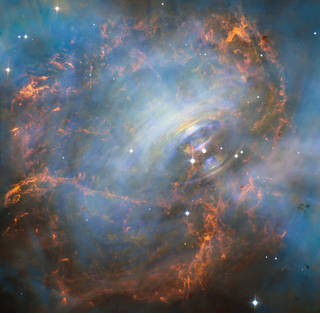
But Betelgeuse is nearly ten times closer than the Crab Nebula. If it explodes before human civilization comes to an end, it will be the nearest supernova in recorded history. Both the explosion itself, and the remaining nebula afterward, would in accordance appear much brighter in the night sky. The visible explosion may be preceded by a surge of neutrinos, which specialized observatories on Earth could detect some hours in advance.
Within a period of a few days, Betelgeuse would abruptly flare up from its normal appearance, and become brighter than the planet Venus. Over the next few weeks it will approach its peak. Most astronomers estimate the peak magnitude to be somewhere between that of a half moon and a full moon; in the vicinity of -12. However, in the case of Betelgeuse, the light is emanating from a tiny point in the sky…a fraction of an arcsecond across, as opposed to 1800 arcseconds. So the light of the supernova may appear even more ‘intense’ to the human eye.
For months afterward, the supernova would shine in broad daylight, and cast brilliant shadows at night. Finally after a year or so, the light gradually begins to fade away. Betelgeuse is close enough to Earth, that the central star may remain visible for several years during this process. As the star continues to get dimmer, the expanding nebula of star dust will become visible in its immediate surroundings. Finally, the central star will become so faint that it vanishes from the night sky forever.
Although Betelgeuse will be gone for good, its nebula is going to remain as a new feature of the winter night sky. Now there will be not just one Orion Nebula, but two! It should appear faintly visible to the human eye, and be quite a spectacular treat in binoculars or a small telescope. And embedded in that nebula will be a very tiny and unimaginably dense neutron star, spinning very rapidly and sending out pulses of radio wave energy.
While supernova explosions emit a massive shockwave into the surrounding space, that surge won’t come anywhere close to reaching Earth. Small amounts of cosmic radiation from the explosion are expected to reach Earth’s upper atmosphere, but it won’t be concentrated enough to do any sort of damage. Probably the greatest risk to humans would happen if they looked at the explosion through a telescope; that much concentrated light emanating from a single point could be dangerous to the eye.
=====
In any case, I will be watching closely over the next several months to see how the brightness of Betelgeuse evolves. Now, if only the skies here in the Pacific Northwest would cooperate for a few nights! -Karl
SOURCES
earthsky.org/space/betelgeuse-dimming-late-2019-early-2020-supernova
britannica.com/place/Betelgeuse-star
www.aavso.org/vsots_alphaori
www.aavso.org/variables-what-are-they-why-observe-them
www.aavso.org/types-variables
skyandtelescope.com/observing/fainting-betelgeuse/
www.space.com/6638-supernova.html
www.space.com/16989-crab-nebula-m1.html
www.forbes.com/2017/03/22/what-will-happen-when-betelgeuse-explodes
Also:
www.maa.clell.de/Scholar/star_evol.html
(good for understanding stellar evolution)
wikipedia.org/wiki/Nuclear_binding_energy
(this is what stops iron from fusing further)